The following insights are part of an occasional series authored by Greg McMillan, industry consultant, author of numerous process control books and a retired Senior Fellow from Monsanto.
Editor’s Note: This is Part 3 of a four-part blog series on smart automation of vessel heating and cooling. Click these links to read Part 1 or Part 2 or Part 4.
In this post I look at how we can eliminate oscillations associated with the discontinuity and changes in phase between heating and cooling and make the response of the secondary temperature loop faster. Most temperature loops tend to develop an oscillation across the split range point during the transition between heating and cooling. The transition creates a discontinuity resulting from the change in utility fluid and the increase in friction and loss of sensitivity in valve seating or sealing.
The use of a vessel PID with external reset feedback and setpoint rate limits on the manipulated valves or flows for cooling and heating can provide directional move suppression to help eliminate unnecessary crossings of the split range point. The setpoint rate limit in the direction of approaching split range point when close to the split range point is set slower. The result is directional move suppression. The vessel PID does not try to change the setpoint faster than the flow will respond by external reset feedback of the Analog output or flow loop process variable (PV). The external feedback of actual valve position or flow can also eliminate the oscillations from deadband and in some cases stick-slip.
Given that crossings of the split range must occur in the transition from heating to cooling, we need to recognize and address the challenges to enable tight vessel temperature control. The worst case is often the transition between steam and water coolant due to the huge difference in temperatures and the creation of steam bubbles in water coolant or water droplets in steam. Steam valves tend to have higher seating and sealing friction due to the higher temperatures and pressures. Hot and cold liquids and tempered water systems offer a much smoother transition than between steam and water coolant and reduce nonlinearities for coil and jacket temperature control.
A jacket or coil temperature loop can isolate the effects of process and valve gain nonlinearity from the vessel temperature loop. Jacket or coil inlet temperature control provides quicker correction of utility disturbances and better prevention of cold and hot spots. Outlet temperature control offers a smoother response by attenuation of mixing disturbances and phase discontinuities. A change in outlet jacket or coil temperature for the same production rate or batch cycle time and same vessel temperature can provide a warning of an increase in heat transfer surface coating or fouling. The jacket or coil temperature control must be fast and responsive to the demands of the vessel temperature controller. See Greg McMillan's ISA book Advances in Reactor Measurement and Control for an extensive view of practical opportunities for designing control strategies to achieve product quality and maximize yield and capacity in different types of fermenters, bioreactors, and chemical reactors.[/dropshadowbox]
Steam does not provide uniform heating in coils or a jacket. Steam typically collects in the top of the jacket and condensate collects in the bottom. Hot spots can develop around inlets. Thermal shock and steam hammer can damage glass lined vessels. The time required to drive steam completely out of the jacket before cooling water is introduced introduces a considerably delay in the control system. Improper trap design or operation can cause condensate buildup.
The addition of hot water instead of steam directly into the coil or jacket provides a more uniform heat distribution, a dramatically smoother transition between heating and cooling, and a more efficient and maintainable system. For rapid heating, the use of direct steam injection heaters and pressurized water as shown in Figure 1 can provide hot water temperatures well above 100oC. If the injection heater has hundreds of small orifices, the bubbles are extremely small and are rapidly and quietly mixed into the water. Variable orifice steam injection heaters are not as quiet and the mixing is not as complete. The use of jacket outlet temperature reduces the possibility of bubbles hitting the temperature sensor.
Insight: The use of hot water instead of steam for heating eliminates the discontinuities and noise in the transition between heating and cooling. A steam injector can be used to provide a smooth and rapid transition from cold water to varying degrees of hot water.

Heat exchangers in the coil or jacket recirculation system are used to provide a tempered water system. This allows the use of colder or hotter utility streams as inputs. As the inputs are to heat exchangers rather than being direct inputs to coil or jacket, temperature extremes are moderated that would cause heat transfer surface coating or product degradation from localized cold and hot spots thermal shock that could crack a glass lining.
Mammalian bioreactors are particularly vulnerable to temperature extremes because of metabolic sensitivity. There is also a greater propensity for localized temperature variations due to less mixing from the reduction in agitation to avoid cell rupture. Mammalian cells, unlike bacterial cells, have membranes rather than cell walls, making them more susceptible to damage.
Insight: Tempered water systems can eliminate cold and hot spots in heat transfer surfaces.
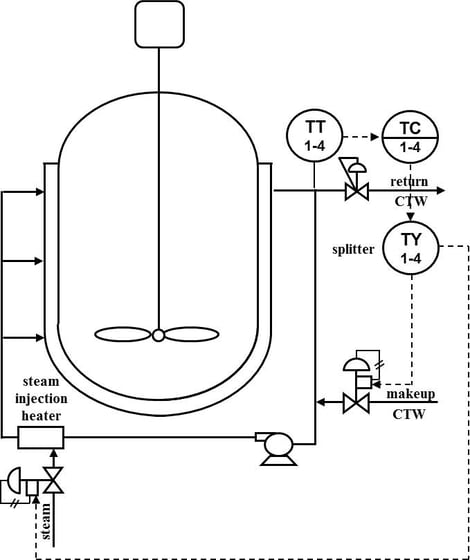
Separate exchangers are used for steam and chilled water. The coil or jacket temperature response to a change in controller output may exhibit a temporary initial change (lead) followed by a ramp (integrating response) before approaching a final steady state value (self-regulating response). The integrating response originates from the recycle of jacket water. For example, an increase in heat exchanger outlet temperature makes a loop through the coil or jackets and comes back to the heat exchanger as an increase in inlet temperature. The ramp rate (integrating process gain) increases as the coil and jacket volume decreases.
A lead in the opposite direction of the response to a change in steam flow can be caused by a temporary change in the condensing rate of steam. This lead causes the temperature to temporarily increase when the steam flow is decreased. There is a thermal lag from the UA of the heat exchanger and an increase in process gain and dead time at low utility flow in the self-regulating. Coil and jacket temperature control by the manipulation of a cooling or heating utility stream to a heat exchanger has a slower and more nonlinear and irregular response than the direct steam injection and blending of hot and cold water in a constant coil or jacket circulation flow.
Insight: The use of heat exchangers in a utility recirculation system can introduce a temporary initial change (lead) followed by a ramp (integrating response) before getting into the heat transfer time constant (lag) of the exchanger volume and heat transfer area.
The coil or jacket exchanger thermal lag can be passed by the manipulation of an exchanger bypass flow creating a faster temperature loop that is easier to tune. A valve position controller (VPC) can be used to reduce cooling or heating liquid utility flow during low loads and to increase temperature loop turndown. Steam heat exchanger bypass control is not used because of steam blowing into the condensate system at low heating requirements.
Insight: The throttling of a heat exchanger bypass flow instead of the cooling and heating flow makes the secondary jacket temperature control faster by eliminating the exchanger thermal lag.
Use a PID with external reset feedback and directional moves suppression to help eliminate unnecessary crossings of the split range point and oscillations from the increased stick-slip near the closed position. Avoid if possible the switch between steam and coolant to the jacket by the use of steam injector or a tempered water system. When heat exchangers are used, the manipulation of a bypass flow instead of a utility flow provides a faster jacket temperature response. A valve position controller can be used to help ensure a minimum utility flow at low loads.
About the AuthorGregory K. McMillan, CAP, is a retired Senior Fellow from Solutia/Monsanto where he worked in engineering technology on process control improvement. Greg was also an affiliate professor for Washington University in Saint Louis. Greg is an ISA Fellow and received the ISA Kermit Fischer Environmental Award for pH control in 1991, the Control magazine Engineer of the Year award for the process industry in 1994, was inducted into the Control magazine Process Automation Hall of Fame in 2001, was honored by InTech magazine in 2003 as one of the most influential innovators in automation, and received the ISA Life Achievement Award in 2010. Greg is the author of numerous books on process control, including Advances in Reactor Measurement and Control and Essentials of Modern Measurements and Final Elements in the Process Industry. Greg has been the monthly "Control Talk" columnist for Control magazine since 2002. Presently, Greg is a part time modeling and control consultant in Technology for Process Simulation for Emerson Automation Solutions specializing in the use of the virtual plant for exploring new opportunities. He spends most of his time writing, teaching and leading the ISA Mentor Program he founded in 2011.