This blog post was written by Jay Werb, technical director of the ISA100 Wireless Compliance Institute.
Industrial wireless instrumentation is rapidly becoming the technology of choice for a growing class of applications. A wireless deployment saves significant costs compared to an equivalent wired installation, resulting in savings of 20 to 30 percent in simple configurations. Cost reductions can be even more compelling in scaled installations or in remote locations. Where wiring is cost prohibitive or infeasible, wireless enables best-practice instrumentation wherever it is needed for efficient and safe industrial operation.
The cost advantages of wireless instrumentation improve with scale. In a wired system, the cost of each additional instrument requires extra wiring and the associated labor, equipment, and maintenance. A wireless system, if designed for scalability, can accommodate additional devices with the same infrastructure and no additional wiring. For the first time, applications with hundreds or thousands of measurement points can be reasonably contemplated.
Until fairly recently, most users and experts viewed wireless instruments as intrinsically inferior to their wired counterparts, with wired instrumentation always being preferred when feasible. As experience with wireless technology grows, this attitude is shifting, with wireless becoming the default user selection for well-proven applications. Today, major users require cost justification for wired instrumentation in applications where wireless has been demonstrated to exceed user requirements.
In Clayton Christensen’s model of innovation, adapted in figure 1, low-cost products initially take a beachhead position with simple applications at the lower end of a market. From that starting point, they work their way up-market. A tipping point occurs when mainstream users discover that the low-cost products can be used in high-end applications. Today, most major users are in the “mainstream adoption” zone, which is shown as an oval to indicate that adoption rates vary from user to user.
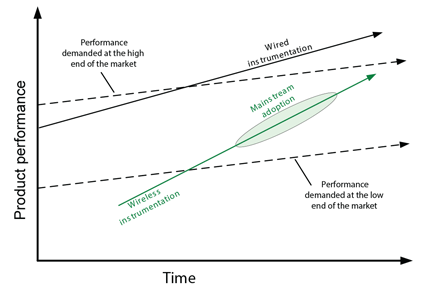
Wired versus wireless instrumentation
Table 1 summarizes the main differences between wired and wireless instrumentation. Some of the listed characteristics, such as fading and interference, relate to radio considerations. Other characteristics, such as battery replacement, relate to energy constraints when wireless instrumentation operates in locations without power.
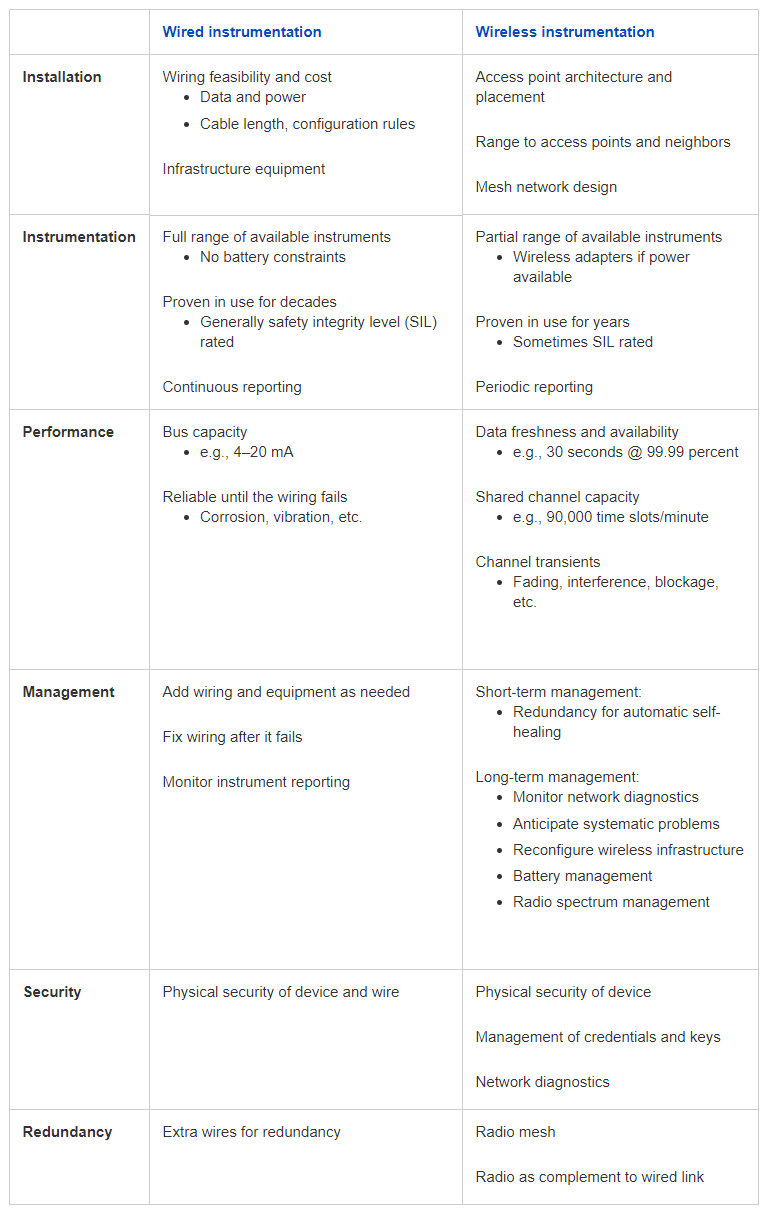
Major advantages of wireless instrumentation include:
- Lower cost, especially when large numbers of instruments are installed.
- Manageability. When wired connections fail, they are typically complete failures that occur without notice. Wireless failures are usually transient, and those transient problems can mostly be avoided by preventative maintenance linked to wireless diagnostics.
- Flexibility. After a wireless system is installed, it is easy to add new wireless instruments and also to report more data from existing instruments using wireless adapters.
- Security. Wireless security extends to the field instrument and does not rely on physical security of the transmission medium.
- Redundancy within a wireless network. Typically, wired instrumentation relies on a single wire to each instrument, with various opportunities for failure. Field experience demonstrates that a redundant wireless channel can be every bit as reliable as a nonredundant wired channel, particularly when wires are long or subjected to challenging conditions.
- Redundancy at the plant level. A wireless system can add redundancy to wired reporting, with the same data reported through wired and wireless channels. Similarly, when field instrumentation is involved in an independent protection layer (IPL), wireless may be an advantage if another IPL uses available wiring.
Disadvantages of battery-powered operation include:
- Battery maintenance. Maintaining the batteries of wireless devices somewhat offsets wireless cost savings. A well-designed wireless solution should ensure that battery replacement occurs in conjunction with an instrument’s general maintenance interval.
- Limited wireless instrumentation. An ISA100 Wireless adapter can convert a wide range of existing instruments to wireless, but only if the wired instrument has the power to operate. In locations where there is no source of external DC power, instrumentation options can be limited. New wireless products are being rapidly released to meet market demand.
- Continuous reporting. In some use cases, it is not technically feasible to sample and report process data continuously under battery power. ISA100 Wireless devices can be configured to report process data frequently in critical applications, with predictable battery life impacts, but only if the sensor has the energy to collect the data in the first place.
Disadvantages of radio operation include:
- Procedural barriers. Wired instruments have been used for decades, and processes for specifying and approving wired systems are well established at user sites. Many of these same users do not have clear processes for approving wireless, particularly when safety credit is involved.
- Statistical nature of radios. Radio performance is statistical by nature, with packet errors and retries being fundamental considerations for any wireless system design. A well-designed wireless system will have plenty of built-in margin, as well as extensive network diagnostics that detect loss of margin even while the system achieves its performance objectives.
- Limited reporting rates. ISA100 Wireless is designed to support reporting as frequently as every 0.25 seconds with a transmission latency of 0.10 seconds in structured configurations. Faster reporting rates are considered (by the major standards) unsuitable for battery-powered operation at this time.
- Spectrum management. Wireless instrumentation shares the radio spectrum with other systems and applications. Spectrum management generally needs to be considered when each new wireless system is installed, and should also be continuously monitored. A well-designed wireless system will have performance margins built in and will include extensive diagnostics to detect loss of margin due to radio interference and other considerations. ISA100 Wireless radio diagnostics include metrics that are specifically intended to detect and blacklist problematic radio channels automatically.
Based on real-world experience with all of these factors, users of industrial wireless are learning that wireless can deliver more than adequate performance for a wide range of applications.
Use cases for industrial wireless instrumentation
Industrial wireless instrumentation is being applied to a wide variety of applications today. Figure 2 represents one way to summarize the major application use cases.
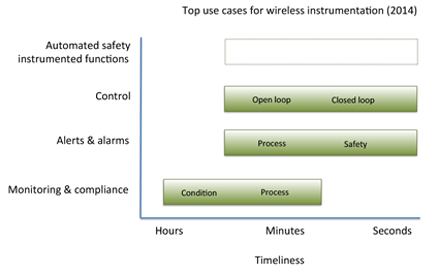
The vertical axis shows types of applications, derived from ISA100 usage classes:
- Monitoring and compliance applications track the status of equipment or a process state, such as temperature or vibration. Monitoring data is archived for subsequent review and may or may not be displayed to operators.
- Alerts and alarms track the status of a process state, such as temperature, or a safety state, such as hydrocarbon gas level. Exceptions are reported to an operator for appropriate action.
- In control applications, wireless is somehow involved in a control loop. “Open loop” means that a user is in the loop; “closed loop” means that the loop is automated. Closed-loop applications are sometimes divided into outer loop and inner loop.
- In an automated safety instrumented function (SIF), a set of equipment is intended to reduce the risk of a specific hazard in an automated safety loop.
The green boxes in figure 2 indicate where wireless instrumentation is targeted, in actual practice in 2014. This is intended as a statement of fact, not to imply that wireless is unsuitable for other applications. For example, ISA100 Wireless was designed for subsecond reporting rates, but subsecond timing is not shown in figure 2 because few wireless instruments today are so configured. Similarly, wireless instrumentation for automated SIF is shown with a dotted line to suggest that it is feasible but not currently being adopted by many users.
Alerts, alarms, and safety
Alerts and alarms merit special mention due to safety considerations. Alarming involves an out-of-bounds condition that is reported to a user or system. Yokogawa Electronics Corporation has reported that more than 50 percent of its wireless instrumentation projects require a 1–10 second update period. Cited applications include gas detection, fire detection, monitoring for operation (power), monitoring for safety (steel), cold temperature monitoring (gas), and tsunami detection. All of these can be considered “alarming” applications.
According to ANSI/ISA-18.2-2009, an alarm is “an audible and/or visible means of indicating to the operator an equipment malfunction, process deviation, or abnormal condition requiring a response.” The essential element of this definition is the response to the alarm.
The term alert is generally used for applications with less rigorous requirements than alarms, for example maintenance alerts where the user response is not specific or time critical.
A “safety-related alarm” designation can be applied to an alarming application that is a candidate for safety credit as an IPL. To simplify approvals, less than 0.9 availability may be claimed, thereby classifying an alarm as a basic process control system. Regardless of an alarm’s classification, high reliability is invariably a key objective.
It is well established that an alarm system must be designed to effectively handle individual alarms during normal operation and handle many alarms during a major plant upset. Wireless has the potential to support many more alarms than has been feasible in the past, so alarm management is an essential consideration in a scaled wireless implementation.
Gas sensing is an important use case for wireless alarming. The requirements vary by application and region. For example, the process safety time of response (t90) for hydrocarbon gas detection as required in IEC60079-29-1 is 60 seconds. In Japan, the time to alarm must be within 30 seconds for a specified concentration.
ISA100 Wireless and the Internet of Things
ISA100 Wireless was designed as an extension of the Internet, building on the IPv6 “Internet of Things” standard. IPv6 is an addressing scheme that allows an essentially unlimited number of devices to be individually addressable. Collections of devices can still be segregated as needed for security and manageability, but with IPv6, addressing considerations are no longer a constraint. Most forecasts suggest that tens of billions of devices will be Internet accessible within a decade. With its use of IPv6, ISA100 Wireless is well positioned to ride that wave.
IPv6 networks use familiar standards-based addressing and can be managed using tools derived from traditional information technology network management tools and systems. Today’s generation of network managers and automation engineers understand IPv6, and so will future generations.
Figure 3 illustrates the flexibility of the ISA100 Wireless universal network in an example mesh network comprised of devices from multiple manufacturers. Some manufacturers use the native ISA100 Wireless protocol, while others use ISA100 objects with different protocols at the same time. The two legacy HART devices, wired to a controller, are simultaneously reporting HART digital data to the ISA100 Wireless network via ISA100 WirelessHART adapters.
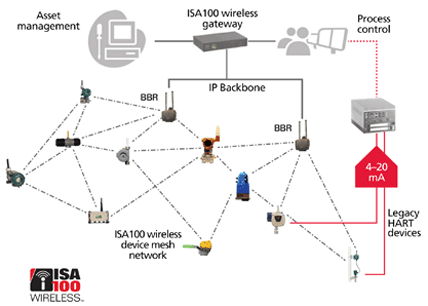
The Internet of Things approach in ISA100 Wireless affects network architecture and the user experience in actual practice.
Figure 4 shows a structured network design that leverages IP. The ISA100 Wireless network is shown as a single cloud or mesh with multiple IP backbone connections. In this configuration, multiple gateways are shown as Internet appliances without a radio. ISA100 Wireless messages flow over the IP network. Networks of this kind are generally rolled out using a structured methodology, similar to the way a Wi-Fi or cellular network is typically deployed.
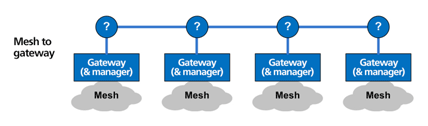
Ready for prime time
Industrial wireless instrumentation is now at a stage where major users are rolling out ISA100 Wireless programs. The “tipping point” for industrial wireless occurs when major users switch from an ad hoc approach to wireless campaigns. In a bottom-up ad hoc approach, small wireless systems are installed one by one to address specific problems, relying on the passion of proactive early adopters. In a top-down campaign, solutions are rolled out to support enterprise strategy. A good process allows for both approaches, with successful ad hoc systems being a laboratory for future wireless campaigns.
Industrial wireless instrumentation is widely considered suitable for monitoring, control, and alarms, including safety alarms. Systems may be deployed using an ad hoc methodology to get started, but users are better served when systems are scaled using a more structured methodology that leverages the Internet of Things. User acceptance of and demand for IP-based scaled mission-critical wireless technology is driving adoption of ISA100 Wireless. ISA100 Wireless is poised to support a fast growth curve with its open design, scalable system and product architectures, and vendor-neutral ecosystem.
About the AuthorJay Werb is the technical director of the ISA100 Wireless Compliance Institute (WCI), where he manages the organization’s compliance and other technical programs. He is also the editor and author of the data link layer (mesh) section of the ISA100.11a standard. Werb has more than 30 years of experience in the computer field, with the past 20 years focused on wireless. He has been the technical founder of multiple technology companies and holds more than a dozen patents. In addition to his work with WCI, Werb is a consultant with AIW LLC, where he assists end users with strategic adoption of industrial wireless instrumentation. He has a B.S. in biology and a master’s degree in management, both from the Massachusetts Institute of Technology.
A version of this article also was published at InTech magazine.